Hunting Higgs bosons: A tribute to Peter Higgs by Lyn Evans
This week, a special tribute to the revered British scientist, Peter Higgs, who died on the 8th of April, aged 94. His friend, Lyn Evans, tells us about the 40-year search for the eponymous Higgs boson: the God particle that provides some of the answers to life, the Universe and everything…
In this episode
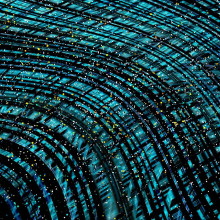
What is the Higgs boson?
Lyn Evans
Lyn - Well, the latter part of the 1960s was a mess. It was more like a whole zoo of particles being discovered all the time. We had, of course, the electron had been discovered by JJ Thomson and at the end of the 19th century, we had the proton and the neutron, and then we had many other kinds of composite particles that we really didn't understand. I came to CERN in 1969, and in January 1970, there was a lecture by Richard Feynman, Nobel Prize winner and a very gifted physicist. He gave a lecture on results that were coming out of Stanford Linear Accelerator Center at the time where they were probing the atomic nucleus of hydrogen and discovering structure inside that proton. There were more fundamental things inside there. And then things started to drop into place. What he was describing, it took a few more years to, to realise that he was describing the quarks. The most fundamental particles that make up the proton, first of all. And then the heavier atoms and the neutron are all made of much more simpler things. So from a zoo and a mess over my career, particle physics has distilled itself down to what is known as the standard model, and produces a very simple picture of something that looked extremely complicated in the 1960s.
Chris - Although people were quite quick to point out that it didn't explain everything. And that's presumably where Peter Higgs comes in and where the planning for the Large Hadron Collider comes in.
Lyn - That's right. So there were basically three families of quarks discovered, and the big problem there was they all had different masses. And then the question is, what was behind all of this? What generated mass? How did they obtain the mass? And it was actually in 1964 that Peter Higgs wrote a paper on what is now called spontaneous symmetry breaking in the electroweak sector. That sounds very complicated, but I was with Peter Higgs about a year ago. We went to have a beer in the pub, and there was nowhere to sit except there was half a table spare. And so we joined a coal miner from Fife and his wife. Peter's, not Scottish, this was in Edinburgh, but he'd been in Edinburgh for many, many years. Peter started to, to tell them a lot of the history of this region of Edinburgh that we were in. And the guy said, you are a very clever man. And I said, he should be. He does have a Nobel prize after all. And then Peter gave them a half hour lecture on spontaneous symmetry breaking in the weak interactions to which they listened intently, but <laugh>, they'll go away with that memory, of course. But it was extremely funny.
Chris - You are there in that part of the 1960s. We know that we've begun to see some order being brought to this what we now call the Standard Model. We know, identified by scientists like Peter Higgs, that there's some gaps there, including explaining why things have the masses that they do. So why did it become apparent that we needed a really powerful particle accelerator to get underneath this problem? Why was that the next step? Was it just a question of, well, we've, we've smashed things together with a certain energy, if we do it harder, we might find even more. Was that the mentality, the thinking?
Lyn - The mentality is if you want to create massive objects, then e equals mc squared. The most famous equation in physics. If you want to create the heavier elements of the standard model, the heavier quarks, you need more energy. But things went in an interesting way. It wasn't straight to the Large Hadron Collider by any means. In 1970 to 1973, there was an experiment at CERN using the tool of the day, which was a bubble chamber. A bubble chamber contains a liquid, super saturated liquid, which when a particle goes through it, it leaves a track. And there was this bubble chamber called Gargamelle, where they made a very important discovery of what were called weak neutral currents. Because there are not only the matter particles, there are not only the quarks and leptons, there are also the force particles. The force particles are basically the photon for electromagnetism, the gluon which is holding the atomic new nucleus together, the strong interaction. Things called the W and Z boson, which are responsible for the weak, very weak interaction. It's weak, but it's very important because that's the way the sun works. That's the way we get life. And these W and Z bosons, because of the experiments on the Gargamelle bubble chamber, were predicted to exist and their mass was predicted. So we actually modified the super proton synchrotron, which I had been working on building, into a colliding beam to smash the nuclei of hydrogen, the protons together to try to create these W and Z bosons. Except that we had to be much more sophisticated and we had to collide protons with antiprotons. Every metaparticle has got its antimatter equivalent, which does not exist in normal life, but we can create it in our accelerators. So we built a machine and we discovered the W and Z bosons. And there was only one thing left to discover, to complete the standard model, and that was the Higgs boson. So that was the Standard Model complete apart from the thing that holds it all together - the particle that creates mass, and that was predicted by Peter Higgs himself a long time ago actually, but couldn't never be found because nobody knew what its mass was. And if you don't know what his mass is, you don't know if you've got enough energy to create it.
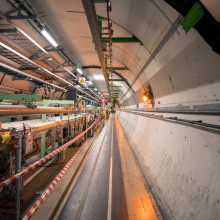
07:23 - How the Large Hadron Collider found the Higgs boson
How the Large Hadron Collider found the Higgs boson
Lyn Evans
Chris - So does that mean then that when the LHC was conceived, because the challenge was, 'can we get at the Higgs boson', You didn't actually know if it would have enough energy to find the Higgs?
Lyn - Yeah, this was the important thing about the LHC I think. First of all, the question was did the Higgs boson exist? Because this is very difficult to explain how the Higgs boson imposes mass on the other particles. It's not actually the Higgs boson, it's the Higgs field. The idea of a field, a gravitational field, which is extremely weak and actually doesn't fit in the Standard Model at all. That's one of the mysteries. But the electromagnetic field, the field of magnetism, for instance, these are all fields, and every field has got a quantum of the field, a particle associated with the field. So for the electromagnetic field, it is the photon, the particle of light which is the quantum of the field. Now, the postulate was there is a field called the Higgs field, which pervades all of our universe, and it is the interaction of particles with the Higgs field that gives them mass.
Lyn - The photon, the particle of light, doesn't interact with the Higgs field at all. So a photon has got zero mass, which doesn't mean to say it's not useful because it brings us light from the sun, it operates our mobile phones. But this Higgs field, the stronger it interacts, the higher the masses of the individual particles. So associated with the Higgs field, there should be the quantum of the field, which is the Higgs boson. So to prove the existence of the Higgs field, we had to create the Higgs boson. That point, all of this is very fine, but nobody had any way of predicting the mass of the Higgs boson. When we designed the large Hadron Collider, first of all, we had experience from the previous proton antiproton collider of how to build it. But we designed it with enough energy so that if the Higgs boson existed, we were bound to find it. We built it with the energy or the mass of the Higgs boson that we discovered, but we built it so that if it existed, we were bound to find it. But the question is, did it exist or not?
Chris - I mean, that's why we're all in a job though, isn't it? That's why we do science, because you think big, you have a theory, you then design experiments to test it. But how did you know that you could make a Higgs boson? What was the physics that led you to believe if we smash protons together, we'll get this mysterious particle that might be the quantum that underpins this field that gives things mass. What was the thinking behind that?
Lyn - The way that these colliders work of course, when you smash protons together. I explained the proton is a composite object. It's like smashing two oranges together. Inside the oranges you've got the pips. So you get an awful lot of junk, but occasionally you get very hard collisions between the pips. And the proton is a little bit like that. Inside the proton there are the quarks. So when you do that, then you produce energy, which then can transform itself into the Higgs boson for a very tiny amount of time, and then it disappears back into the Higgs field. So the idea is you convert energy into mass by accelerating these particles up to very high energies and colliding them together, and you look at the debris of the collisions.
Chris - How did you know what to look for? Because if you don't know something exists, how did you know what the footprints of the Higgs boson transiently popping into existence in that debris that you created from those collisions? How would you know what it looked like?
Lyn - If it existed that was rather easy to predict the decay modes that it would have. There are some decay modes, even though they're extremely rare. One very clean channel, for instance, is the Higgs decays into two photons, and the two photons have to go back to back to conserve energy and momentum. So you'd see two photons coming out of a collision that you would measure their energy, and the sum of their energy would be the mass of the Higgs boson. So that was one way. And there are other signatures as well where you get four electrons coming out. So these were predictable decay modes, so we knew what to look for and to build the detectors to detect them actually.
Chris - And how quickly after kicking off with the LHC was it apparent that you were into the regime you needed and that you were beginning to see promising detections that suggested you were onto something and that we were going to get the Higgs?
Lyn - So once we switched on the LHC, then the big issue was getting the luminosity. The luminosity is the number of collisions per second that we could produce. Because the Higgs is so very rare, we would've to do many, many things to see the Higgs Boson. And when we switched on the LHC, actually, one thing you may or may not remember is that as when we were commissioning the LHC, we had an accident which cost us about a year when one of our superconducting joints failed and produced a big mess. Anyway, we had to fix that, but that gave us an extra year to get everything ready. So when we actually switched on the LHC it came on very quickly. And the first real year of operation that we were in a regime where we could expect to see first signs of the Higgs. And there was a seminar at CERN on July 4th, 2012. This seminar showed there are two detectors on the LHC, which are capable of seeing the Higgs boson, they're called ATLAS and CMS, two huge collaborations that are taking data off the machine. And on that day, neither of the collaborations knew what the other one was going to show. They showed data that showed a small peak in the mass spectrum, at 125 GeV. Any one of those detected, the signal would've been very, very interesting, but not sufficient. But when you put the two together in real time in the lecture theatre and Peter Higgs was present in that seminar, then it was absolutely obvious that the Higgs boson had been discovered because the two results just matched perfectly. So that was a great day that I will never forget actually.
Chris - And was presumably Peter Higgs either awe struck or did he sit there looking smug? What was the reaction of the future Nobel Prize winner when these results went up?
Lyn - Peter is a very modest guy. He's a theoretical physicist. He just could not imagine the efforts that the whole world community had put into finding his particle. He did say, I thought I would never ever see this in my lifetime. And that's imprinted on a t-shirt I have now.
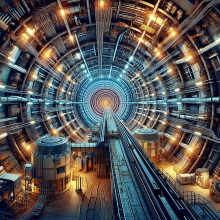
14:16 - What comes after the Large Hadron Collider?
What comes after the Large Hadron Collider?
Lyn Evans
Chris - It is an awesome machine though, the LHC. I remember going to a lecture when it was being commissioned and beginning to run up and someone was saying, we are able to guide just handfuls of protons within a gnats whisker - that's not an SI unit, of course - the speed of light and then slam them together. And they're meeting, despite taking this huge journey of tens of kilometres underground, they're meeting on a path which would fit inside the numerical zero on a small coin where it says the value of the coin. And I just thought it is absolutely incredible that you're able to guide these tiny particles around this massive journey and then bring them into collision paths in such a tiny amount of space.
Lyn - Yeah, the analogy that I use, which is not, not easy to check in the UK, but if you take a one Euro coin, then the Iberian Peninsula on that one Euro coin is the size of the aperture that we impose on guiding these beams around the LHC so that there is no, what we call background in the detectors, there are no junk particles coming in. And when the beams collide, of course, the size is much smaller than the human hair, and we have to get them colliding head on. And not to mention the fact that guiding these beams around on their orbits requires superconducting magnets, which are cooled to 1.9 degrees Kelvin, 1.9 degrees above the absolute zero. And the temperature of deep space is 2.7 Kelvin. So we are lower than that. So the whole thing, when I step back, I wonder how we manage to do that? It just looks awesome. When you're in the middle of it, it not at all <laugh>
Chris - People say though, don't they? Because obviously you say, how do we manage to do that? And there are plans for an even bigger collider coming, and we'll talk about that perhaps in a minute. But some people say, why are we spending, it would've cost a few billion for the LHC, more than 10 billion for what's coming next we anticipate, but there must be some fringe spinoffs from this. There must be in the same way that the space race by presenting us with the scientific equivalent of Everest, creates a challenge that we have to surmount that then leads to enormous spinoffs. So are there enormous spinoffs that have come from the LHC that means that life for the average person on Earth has improved as a consequence. They're not just now the beneficiary of billions of pounds worth of investment to discover the Higgs boson. They're also benefiting because of X.
Lyn - First of all, let me say that it's only possible to do something like this by massive collaboration between countries. And there are 21 member states of CERN, which are contributing to the budget. And that's the only way we can build something like that. As far as spin up is concerned, of course we have to push technology to the limit everywhere, absolutely everywhere. And it's not only super connecting magnets, which are used in MRI scanners in hospitals and things. The analysis that we need to do on this data, that is the worldwide web which CERN invented in the late eighties, early nineties. It's not in the general domain yet, but there is a massive infrastructure which is much more powerful than the web, where the collaborations analyse this huge amount of data coming out of the LHC, in their universities, wherever they are in the world. And of course there is the educational value of CERN. We have massive numbers of students coming and doing their PhDs or even technicians learning their trade here. CERN is the kind of scientific university of Europe.
Chris - The next generation of collider announced recently that there are plans afoot to try to build the Future Circular Collider, three or four times bigger than what we have already. Is that based on the same rationale that we need more energy and so we just build a bigger one and just see what happens? What's the target that they're going for with that? Because you were saying the Standard Model, we now can put that together. We have a good understanding of how all the parts relate. So what are the big questions that you'll solve with an even bigger collider?
Lyn - For the LHC, we had a clear objective, which was to find the Higgs boson. There are many other things that have come out of the LHC apart from the Higgs boson, but there was a clear objective. For the future, there is no clear guidance. That's much harder, much harder to justify a huge expenditure just to see what's there. And that's the quandary we are in now. The circular machine is not the only one. There are other options which would allow us to study the Higgs boson. I explained that when we collide the protons together, we get a whole lot of mess and we get very occasional collisions with Higgs bosons, which are very difficult to analyse. There are ways of producing them in a much cleaner way, which would require a new machine, either this big circular machine or other means like linear colliders or whatever. There are three or four options on the table of how to refine our measurements of the Higgs boson and to go further. But there are considerations. Cost of course is a really important thing, but also power consumption is now becoming a serious issue. The bigger the machine, the more power we consume. And in this day and age, it is not cheap anymore. So all of these things have got to be taken into consideration before a decision and the decision will not be taken, probably not, in the next 10 years of what really to build next. We are now upgrading the LHC to produce high luminosity, so more collisions per second so that we can accumulate more data, get more precision, and that upgrade is being done over the next two years. And then the LHC will probably run for another 20 years after that. So what we are looking at in these other options of which one is this big circular thing, is what is the best way forward? There are still unknown problems. It's not just for finishing the Standard Model. We still do not know what is the subtle asymmetry between matter and antimatter, which means that our universe is made of matter, not antimatter. We do not understand dark matter. Dark matter is something which comes from basically astronomical observations. And even more mysterious dark energy. There are still a huge number of questions out there that we don't understand the answers to, and they're being attacked in many ways. One of the ways of course is to probe the very high energy frontier, but we have to be very conscious that there are only limits that society is prepared to support in building just bigger and bigger things.
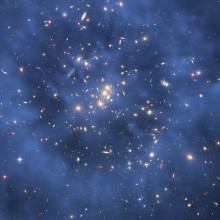
The big unanswered questions in particle physics
Lyn Evans
Chris - Do these other entities like dark matter and dark energy, are they using the Standard Model for their existence or are there bolt-ons that go with our Standard Model, which then explain them? So when we say the Standard Model is complete, is it complete but there are extra bits that can be bolted on the side? Or are they just using what we already know about and it's just we need to find out how they use what we already know about, but in a novel way.
Lyn - The Standard Model is complete in understanding the particles and their interactions that we have. But for instance, gravity doesn't fit into the standard model. Gravity is the oldest force that we know about, but the least that we know, the most mysterious. It's 23 orders of magnitude weaker than the strong forces of the Standard Model. There have been many attempts to make a theory of what's called quantum gravity, which would then integrate gravity into the Standard Model. So that's one thing. Dark matter and dark energy are totally mysterious. We don't have a clue what they are. One theory for dark matter was a very elegant one where, just like we have a mirror world of of matter and antimatter that was predicted, there should be a mirror world of supersymmetry that each of the particles that we know would have a mirror world of particles with similar properties, which we could create in our accelerator. And the lowest mass particle in that supersymmetry could be the dark matter. Now the LHC has discounted that, so it's not only discovered the Higgs boson, it's made one contribution to eliminate what was a very attractive explanation of dark matter. So we are back to square one with dark matter. Dark energy is even more mysterious. It looks like at very large distances there is anti-gravity. And dark matter and dark energy make up about 90, 95% of our universe, we understand 5%, there is 95%, which is a complete mystery to us today. And that's a pretty sobering thought.
Chris - It is indeed. Now, when you are not thinking about these sorts of challenges, which are going to keep physicists very busy for a very long time, what do you like doing to unwind?
Lyn - To unwind <laugh>? Well, when I was younger, I did a lot of sport, of course, I played a lot of rugby, which is a subject, which I do not wish to talk about at the moment. Now, of course, I'm my age, I can't play rugby or squash or anything like that. I play golf which is good in this region. You can only play golf for about eight months of the year because of weather conditions. I've stopped skiing now, but I was skiing for 40 years, which was great. So mostly I sport, but also, I read a lot, I chair committees. One of the most interesting committees that I chair is in the European Southern Observatory, where the headquarters are in Munich, but the telescopes are in Chile, and are building a thing called the Extremely Large Telescope. And I chair their advisory committee. This telescope, you know, any normal telescope we'd have a mirror. The biggest mirror on a telescope is eight metres or something like that. The Hubble has got an even smaller mirror of I think six or even smaller. This telescope has a mirror 38 metres in diameter. Not a single mirror, but a composite thing. So, I do things like that and there's a few other projects as well that I help with. So, I try to stay active and I quite enjoy it. It's good to be able to give advice without having the responsibility on your shoulders.
Chris - A bit like grandchildren. Then people often say they like grandchildren because you can give 'em back. But <laugh> in closing, Lyn, what scientific question would you really like to see answered if one could be answered in your lifetime?
Lyn - Well, for me it's very clear: what is dark matter? I mean, it's absolutely sure that it's there, that there are several different ways of observing its existence, but nobody has got the slightest clue what it is. That's the one, that's the first question that I would like to see answered.
Comments
Add a comment